Imagine a world where computers are made from human brain cells, consuming a fraction of the energy used by current systems. This isn't science fiction—it's happening now. Swiss company FinalSpark has created the Neuroplatform, a groundbreaking biocomputing system that uses tiny clusters of human neurons to process information.
Scientists even rent these platforms for a couple of dollars a month, opening up new frontiers in artificial intelligence and sustainable computing, revolutionizing how we approach AI and computing. But what exactly is this Biocomputing, and how can it help in present AI technology? Let's delve into more of the innovation and use cases.
The Dawn of a New Era in Computing
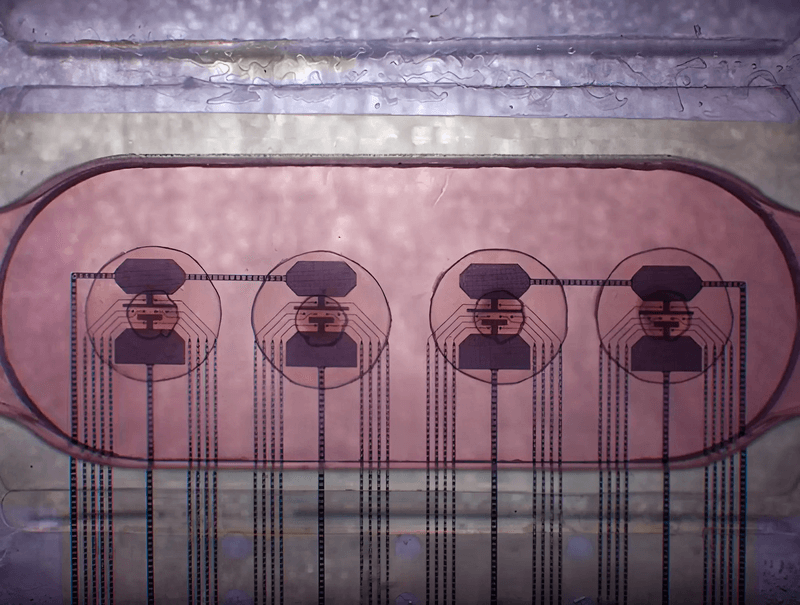
In a groundbreaking development, scientists are exploring the use of human neurons to create living computers. This innovative approach, known as biocomputing, aims to address the growing energy demands of artificial intelligence systems.
Swiss company FinalSpark has taken a significant step in this direction with their "Neuroplatform," a computer platform powered by human brain organoids. This platform is now available for scientists to rent over the Internet for $500 per month, making it the first publicly rentable system of its kind.
Key Features of FinalSpark's Neuroplatform:
Uses processing units with four spherical brain organoids each
Each 0.5-millimeter-wide organoid is connected to eight electrodes
Organoids are stimulated electrically and exposed to dopamine
The setup mimics the human brain's natural reward system
Organoids are trained to form new pathways and connections
Fred Jordan, co-founder of FinalSpark, states,
"Our principal goal is artificial intelligence for 100,000 times less energy" than current AI training methods.
This ambitious target highlights the potential environmental benefits of biocomputing.
The Neuroplatform's organoids are live-streamed 24 hours a day, allowing researchers to observe their behavior in real-time. Jordan explains, "The challenge is to find the appropriate way to get neurons to do what we want them to do."
Research and Collaboration:
34 universities have requested access to FinalSpark's biocomputers
Scientists from institutions like the University of Michigan and the Free University of Berlin are already using the platform
Research focuses on various aspects of biocomputing, including developing organoid-specific computer language and AI learning models
Despite the progress, challenges remain. The organoids currently have an average lifespan of around 100 days, though this is a significant improvement from initial experiments. FinalSpark has also streamlined its organoid production process, with their facility housing between 2,000 and 3,000 organoids.
While other forms of biocomputing are being explored, such as cellular and fungal computing, Jordan remains confident in the potential of human neurons.
He asserts "Human neurons are the best at learning," highlighting the unique advantages of this approach.
As research continues and technology advances, the use of human brain organoids in computing may revolutionize the field of artificial intelligence, potentially offering a more energy-efficient and sustainable alternative to traditional silicon-based systems.
What is BioComputing?
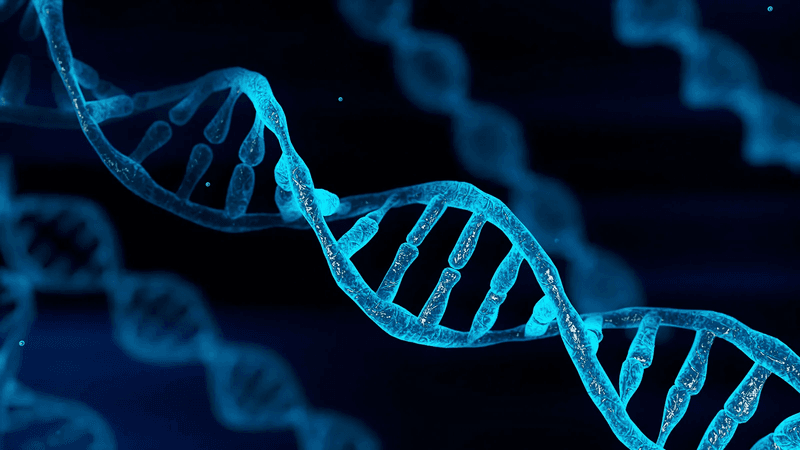
Biocomputing is an innovative field that merges biology with computer science, using biological systems or components to perform computational tasks. This emerging technology uses the power of living organisms or their parts, such as cells, proteins, or DNA, to process and store information. Unlike traditional silicon-based computing, biocomputing leverages the natural information processing capabilities of biological systems.
Key aspects of biocomputing include:
Use of biological materials: Using living cells, organoids, or biomolecules as computational units
Biomimicry: Mimicking natural biological processes for information processing
Integration with traditional computing: Often combining biological elements with conventional electronic systems
Interdisciplinary approach: Bringing together biology, computer science, engineering, and other fields
Biocomputing systems can take various forms:
Cell-based computing: Using living cells, such as bacteria or human neurons, to perform computations
DNA computing: Utilizing the information storage capabilities of DNA molecules
Protein-based computing: Leveraging the folding and interaction of proteins for information processing
Organoid computing: Using lab-grown mini organs, particularly brain organoids, for complex computations
These systems work by manipulating biological processes to represent and process data.
For example, in DNA computing, information is encoded in DNA sequences, and computations are performed through biochemical reactions. In cell-based systems, cellular responses to stimuli are used to process information.
Benefits of Biocomputing
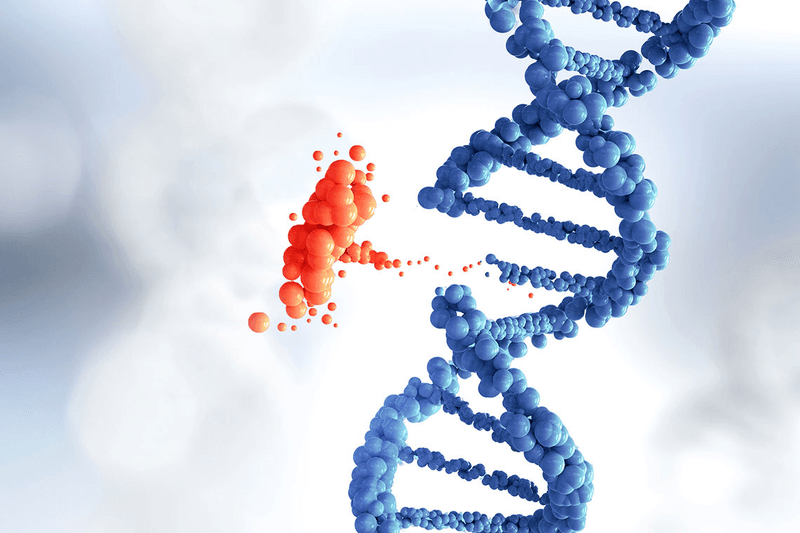
Biocomputing offers several potential advantages over traditional computing methods:
Energy Efficiency: Biological systems are inherently energy-efficient. Biocomputers have the potential to perform complex computations with significantly less energy consumption compared to traditional electronic computers.
Parallel Processing: Many biological systems naturally perform multiple operations simultaneously. This parallel processing capability could lead to faster computation for certain types of problems.
High-Density Information Storage: Biological molecules like DNA can store vast amounts of information in a very small space, potentially surpassing the storage density of current electronic systems.
Self-replication and Self-repair: Some biocomputing systems, especially those based on living cells, have the ability to replicate and repair themselves, potentially leading to more robust and long-lasting computational systems.
Environmental Interaction: Biocomputers can directly interact with and respond to their environment, opening up possibilities for applications in environmental monitoring and bioremediation.
Integration with Biological Systems: Biocomputers could more seamlessly interface with living organisms, opening up new frontiers in fields like personalized medicine and biosensing.
The Role of Human Neurons in Computing
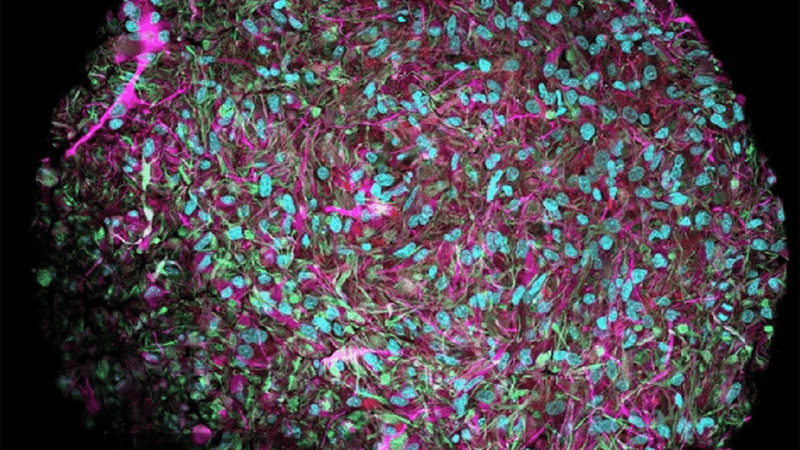
Human neurons are emerging as a groundbreaking component in the field of computing, offering a unique approach to information processing that diverges from traditional silicon-based systems. This biological computing method, known as biocomputing, leverages the natural capabilities of human brain cells to create more efficient and potentially more powerful computational systems.
Key advantages of using human neurons in computing:
Energy efficiency: Potentially reducing power consumption by a factor of 100,000 compared to current AI systems
Adaptability: Mimicking the brain's natural learning processes
Complexity: Capable of forming intricate neural networks similar to those in the human brain
How does Neuroplatform Work?
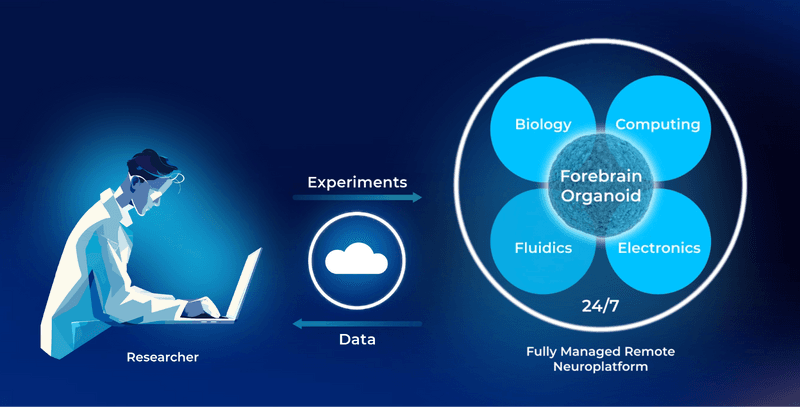
Neuroplatform, developed by FinalSpark, is a pioneering biocomputing system that utilizes human brain organoids as its core processing units. This innovative platform integrates biological components with traditional computing infrastructure to create a hybrid system capable of performing complex computations.
The key components and processes of Neuroplatform include:
1. Brain Organoids:
Miniature clusters of lab-grown human neurons
Each organoid is approximately 0.5 millimeters in diameter
Contain around 10,000 living neurons
2. Processing Units:
Each unit hosts four spherical brain organoids
Connected to eight electrodes per organoid
3. Electrode System:
Provides electrical stimulation to the neurons
Acts as an interface between the organoids and conventional computer networks
4. Neural Training:
Neurons are selectively exposed to dopamine, mimicking the brain's reward system
Electrical stimulation combined with dopamine rewards encourages the formation of new neural pathways
5. Data Processing:
The trained organoids process information in a manner similar to biological neural networks
Potential to perform functions analogous to CPUs and GPUs in traditional computing systems
Neuroplatform's approach aims to harness the natural learning capabilities of human neurons to create a more efficient and adaptable computing system. By combining biological elements with conventional computing technology, this platform represents a significant step towards realizing the potential of biocomputing in artificial intelligence and beyond.
While still in its early stages, Neuroplatform and similar biocomputing systems could potentially revolutionize our approach to information processing, offering new solutions to complex computational challenges while significantly reducing energy consumption
Ethical Considerations: The Bioethical Debate
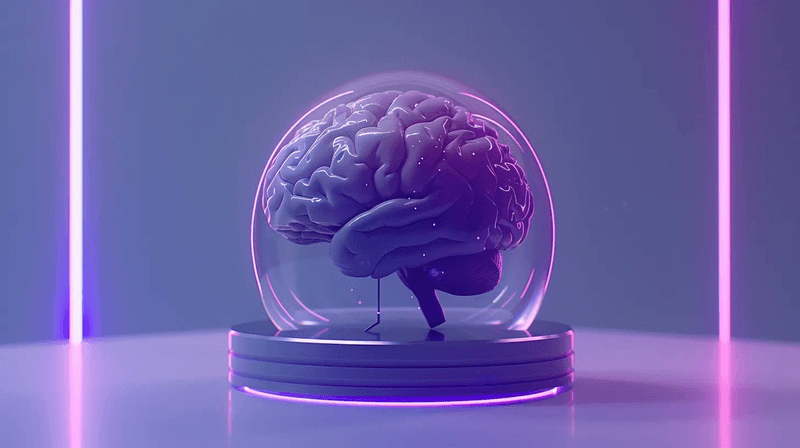
The emergence of biocomputing, particularly the use of human brain organoids in computational systems, has sparked a significant bioethical debate. This new frontier in technology raises complex questions about the nature of consciousness, the ethical use of human tissue, and the potential implications of creating "living" computers.
Key ethical considerations include:
Consciousness and sentience: The primary concern revolves around the potential for mini brains to develop some form of consciousness or sentience.
Rights and moral status: If organoids develop cognitive capabilities, what rights or moral status should they be afforded?
Informed consent: Questions arise about the ethical procurement of human cells for creating these organoids.
Potential for misuse: Concerns about the potential misuse of this technology for unethical purposes or unintended consequences.
The Consciousness Debate:
The most pressing ethical question is whether these organoids could potentially gain consciousness. While there is currently no evidence of consciousness in lab-grown organoids, the possibility cannot be entirely ruled out as the technology advances. This uncertainty has led to calls for careful monitoring and ethical guidelines for research in this field.
Fred Jordan, co-founder of FinalSpark, acknowledges the gravity of these ethical questions, stating that he is "seeking philosophers and researchers with the cultural background to help us answer these ethical questions."
This highlights the interdisciplinary approach needed to address these complex issues.
Regulatory Challenges:
The unique nature of biocomputing presents challenges for existing regulatory frameworks:
How should these "living computers" be classified?
What guidelines should govern their development and use?
How can we ensure responsible innovation while not stifling progress?
Balancing Progress and Ethics:
While the potential benefits of biocomputing are significant, particularly in terms of energy efficiency and computational power, these must be weighed against ethical considerations. Researchers and ethicists argue for:
Transparent research practices
Ongoing ethical review processes
Development of specific guidelines for biocomputing research
Public engagement and discussion on the implications of this technology
As biocomputing continues to advance, it's crucial that the scientific community, ethicists, policymakers, and the public engage in ongoing dialogue. This will help ensure that the development of this technology proceeds in a way that respects ethical boundaries while harnessing its potential benefits. What do you think? Let us know in the comments.
Future of Biocomputing: Challenges and Opportunities
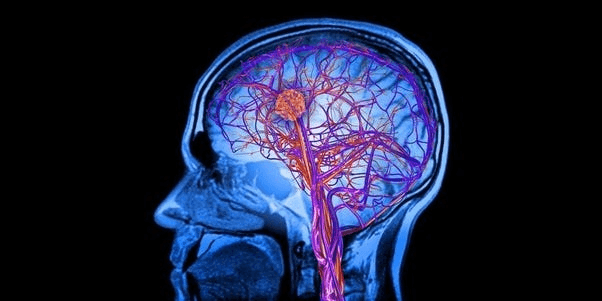
Biocomputing, particularly the use of human neurons in computational systems, represents a frontier in the world of information processing. This emerging field offers exciting possibilities for more efficient and powerful computing, but it also faces significant challenges that must be addressed for its potential to be fully realized.
Opportunities:
The primary driving force behind biocomputing is its potential for drastically reduced energy consumption.
As Fred Jordan, co-founder of FinalSpark, states, "Our principal goal is artificial intelligence for 100,000 times less energy" than what's currently required generative AI models on the internet.
This energy efficiency could revolutionize the AI industry, making advanced computational tasks more sustainable and accessible.
Beyond energy efficiency, biocomputing offers unique advantages:
Environmental responsiveness: As Ángel Goñi-Moreno suggests, cellular computers could facilitate bioremediation. He notes, "You can't just throw a computer into a lake and have it tell you the state of the environment," highlighting the potential for biocomputers to interact directly with their surroundings.
Versatility: Different biological systems offer various computational capabilities. For instance, Andrew Adamatzky's work on fungal computing demonstrates potential for "learning, reservoir computing, pattern recognition, and more."
Integration with existing technologies: Some biocomputing approaches, like fungal computing, offer advantages in "environmental resilience, cost-effectiveness and integration with existing technologies," according to Adamatzky.
Challenges:
Despite its promise, biocomputing faces several significant hurdles:
Standardization: There's currently no standardized manufacturing system for biocomputers, making large-scale production and implementation challenging.
Lifespan: The biological components have limited lifespans. For example, FinalSpark's organoids survive for an average of about 100 days.
Ethical considerations: The use of human neurons raises complex ethical questions on whether the brains can gain consciousness and sustain life.
Technical challenges: Researchers are still working on fundamental aspects of biocomputing on how they can use human neurons to do what we want.
The potential impact of biocomputing extends beyond just more efficient AI. It could lead to new paradigms in computing, enabling tasks that are currently impossible with silicon-based systems. As this field evolves, it promises to not only change how we process information but also how we interact with and understand biological systems.
Conclusion
The emergence of biocomputing, particularly using human neurons, marks a revolutionary leap in technological innovation. FinalSpark's Neuroplatform, with its brain organoids connected to electrodes and stimulated by dopamine, showcases the incredible potential of this field.
The system aims to drastically reduce energy consumption in AI processing by up to 100,000 times while mimicking the human brain's learning capabilities. This groundbreaking technology represents a significant shift from traditional silicon-based computing.
With ongoing research and collaboration across multiple universities, we're on the brink of a computing revolution that could transform AI and our approach to information processing.
Also, Neuroplatform has live-streamed organoid behavior 24/7 for real-time observation and learning of the field to the world. As we continue to explore the potential of living computers, we edge closer to a future where human neurons could power our most advanced computational systems, potentially reshaping the world of artificial intelligence and sustainable computing.
FAQs
1. What is the CPU equivalent to the brain?
The brain is equivalent to a massively parallel CPU, with each neuron acting as an advanced non-linear processor, integrating tens of thousands of inputs simultaneously. This makes the brain far more complex than a typical multi-core computer.
2. What is the computer chip that can replicate the human brain?
DeepSouth is the first supercomputer capable of simulating human brain networks, performing 228 trillion operations per second, replicating the function of neurons and synapses on a massive scale.
3. How powerful would a brain be as a CPU?
The human brain can perform the equivalent of an exaflop (a billion-billion operations per second) while consuming only 20 watts of power, showcasing its incredible efficiency as a CPU.